How do mesh-grids stack up with mini-grids?
In this blog, we’ll take a deeper look at how mesh-grids compare against mini-grids for varying sizes of off-grid communities. These insights are driven up by recent updates to Okra’s Network Planner software.
But first, a quick recap of how mesh-grids work and the different kits they can be built with.
Mesh-grids enable neighbouring households with solar PV and batteries to interconnect and share power autonomously via low voltage transmission lines. With both DC and AC output, this design increases grid uptime and energy availability, thereby providing a cost-effective and scalable alternative to AC mini-grids.
Okra currently offers five kits which can be used to construct a mesh-grid:
- Spoke Kits → no solar PV or battery, just an Okra Pod which draws excess energy from neighbouring households
- XS, S, M & XL Kits → all with solar PV and lithium-ion batteries

As mesh-grids have entered the market, one common perception is that they only make economic sense for the very last-mile, ie. sparsely populated communities that are completely isolated from larger settlements.
But does this perception hold up to closer analysis?
Previously, it was only possible to compare a mesh-grid and mini-grid on a site by site basis by spending hours on manual project analysis, or else by sacrificing accuracy by making back-of-the-envelope estimations. Consequently, it was difficult to draw any generalizable conclusions regarding the place of mesh-grids in the rural electrification market.
Whereas our previous blog on mini-grids vs mesh-grids focused on summarising the key differentiating factors between these two technologies, this blog will compare simulations across a wide array of sites and assumptions; all made possible by updates to Okra’s Network Planner software.
But before we dive into the comparisons, what about solar home systems (SHS)?
We believe the ability to distribute power in mesh and mini-grids makes both of these technologies more robust than SHS. Whilst SHS are typically limited to low-power applications, mesh-grids and mini-grids provide productive power so that individual users can use much more than the 200Wh/day average daily load that has been observed in Africa [1], enabling them to grow their productive use of energy over time. To us, this makes both mesh-grids and mini-grids more compelling than SHS.
Four real site comparisons provide insight into the application of mesh-grids
Let’s use Okra’s Network Planner to evaluate several different sites, starting from a sparse village and working our way up to larger communities.
First, what else should we know about these cost comparisons?
The two main variables we consider when specifying a network are:
- Energy capacity, and
- Simultaneous peak load rating
Here’s an example of this kind of specification:
- Avg. daily load: 250Wh
- Simultaneous avg. peak load: 150Wp
The energy capacity of the network above (250Wh/home) is the average amount of energy available per home per day while maintaining a certain level of system uptime throughout the grid. Some homes may be expected to consume more or less than others. This variable primarily affects the amount of panel and battery capacity required in the network, and it affects both mesh-grids and mini-grids similarly. We have chosen 250Wh as a starting point because it is slightly above the average daily load that has been observed in Africa thus far [1].
The average simultaneous peak load (150Wp above) is the average maximum power that could be drawn by every home in the network at the same time. For instance, 150Wp could be achieved via every single home using 150W at the same time, or through 50% of the homes using 300W while the other 50% use 0W. In either case, the distribution lines of the AC mini-grid would have to carry an amount of current proportional to the average power drawn by the network, and consequently an increase in the average simultaneous peak load will cause a corresponding increase in the cost of the AC distribution network. As a result, this variable primarily affects the cost of AC distribution required for a mini-grid while it has little effect on the cost of a mesh-grid. We have assumed 150Wp as a starting point because at this load rating, the AC distribution network can remain relatively lightweight, with the potential to be scaled up over time if necessary.
The three main levels of AC distribution considered in our model are as follows:
- Single-phase low-voltage (230V)
- Three-phase low-voltage (400V)
- Medium voltage (11kV)
Each step up in the distribution infrastructure increases the power rating of the network but brings an increase in cost along with it.
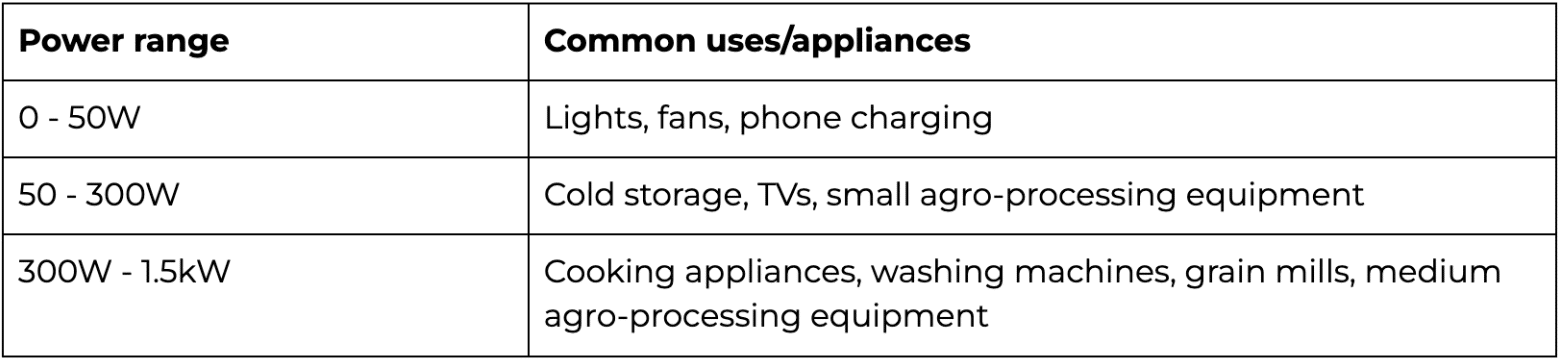
Fig. 2: Examples of common uses of energy by power range. Actual power consumption may vary based on the specific appliance used.
In order to make an “apples to apples” comparison for a given specification, PV capacity and battery autonomy are designed to be approximately the same between mesh-grids and mini-grids. We know that some mini-grid developers prefer to undersize their battery storage and sacrifice reliability, in which case the cost per connection would be lower. But the cost would also be lower for mesh-grids if we were using a lower battery autonomy, so the difference in cost should be preserved regardless. Of course, these costs are estimates, and the real engineered costs may differ. We are experts on mesh-grid costs, and we are making the best estimates we can for mini-grid costs, erring on the conservative (i.e. generous to mini-grid) side.
Additionally, the cost estimates from the Network Planner only account for the bill of materials. For mesh-grids, this value encompasses all costs. However, for mini-grids, there are additional costs in project planning, permitting, and licensing that are estimated to make up over 5% of the total project cost, along with delaying the project delivery time. These additional costs are not included in our cost estimates for mini-grids, but they should be considered as part of the holistic project cost.
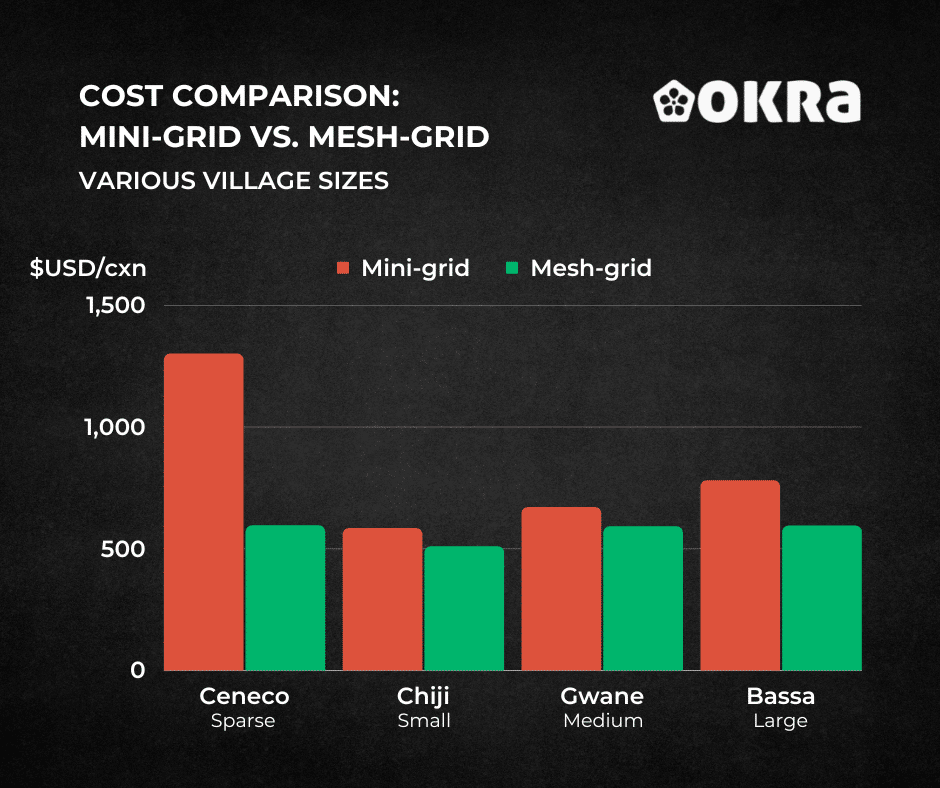
Fig. 2b — Summary of cost comparisons between different village sizes
A sparse community – Ceneco
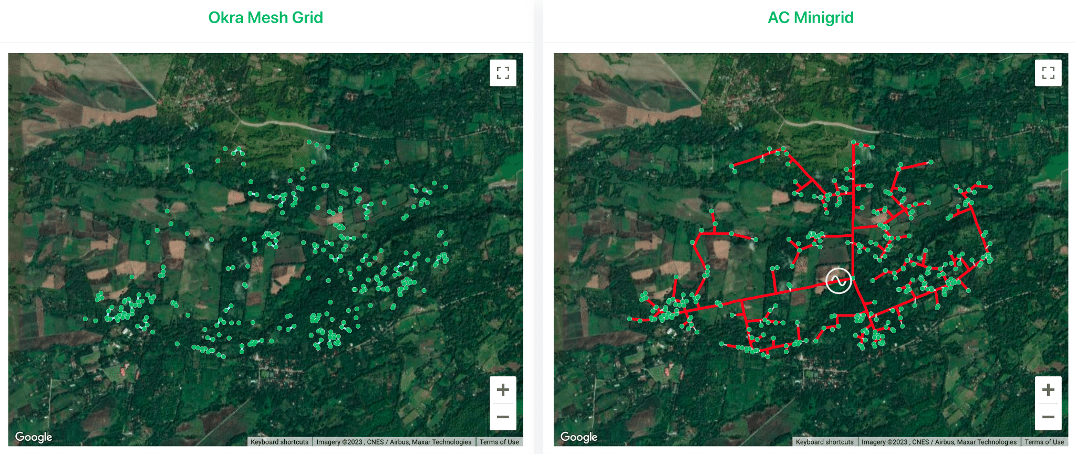
Fig. 3a — Automatically generated distribution mappings of mesh-grid and mini-grid. The mesh-grid removes long cable runs while still allowing energy sharing between clusters of homes, enabling the electrification of sparse communities.
- # households: 323
- Area: 2.94 km2 (110 households / km2)
- Avg. daily load: 250Wh
- Simultaneous avg. peak load: 150Wp
- Cost comparison: ~$596/cxn for mesh-grid vs ~$1300/cxn for mini-grid
The numbers speak for themselves here. Because the spread of the village is so wide, medium voltage (MV) distribution would be required for the mini-grid and when this is multiplied by the long runs of distribution lines, the cost skyrockets. We’re not exactly sure if this is the way a developer would connect this village in practice, but even if a developer used multiple low-voltage (LV) power stations to electrify the site, the distribution cost would remain high due to the long cable runs required.
Obviously a site like this would not be a good candidate for a mini-grid, but it is useful as an illustration to show how the cost blows up when a mini-grid is deployed in a sparsely populated area. In contrast, the mesh-grid cost per connection remains similar regardless of the density.
Small community, single-phase mini-grid – Chiji
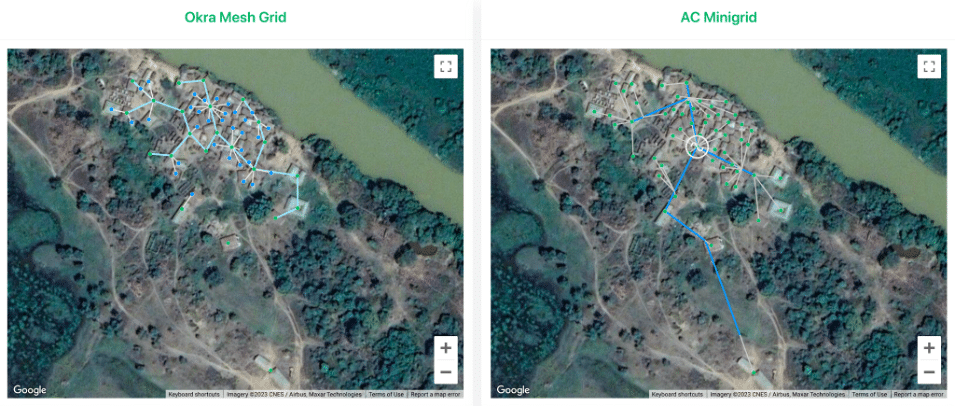
Fig. 3b — Automatically generated distribution mappings of a mesh-grid and mini-grid. The density of this community results in a drastic reduction in the cost of AC distribution.
- # households: 56
- Area: 0.0285 km2 (1965 households / km2)
- Avg. daily load: 250Wh
- Simultaneous avg. peak load: 150Wp
- Cost comparison: ~$509/cxn for mesh-grid vs ~$584/cxn for mini-grid
Because this site is small and densely packed, the AC distribution network consists only of short, single phase power lines, making the cost of the mini-grid comparable to that of the mesh-grid. However, the mesh-grid also capitalises on the community’s density to share assets between neighbouring households efficiently, making it even more cost-effective.
Should the community need to scale its energy use over time, both a mesh-grid and mini-grid provide a cost-effective pathway to doing so, since with a community of this size, a mini-grid would not require MV distribution even at higher power ratings.
While the difference in cost of materials is small, and both technologies offer a path to scale-up here, the added overhead of an AC mini-grid could tip the scale in favour of the mesh-grid. AC mini-grids require more up front work on project planning, permits, and licences when compared with mesh-grids, which could make a mesh-grid the more effective use of resources for a last-mile community such as this.
Medium-sized community, low-voltage mini-grid – Gwane
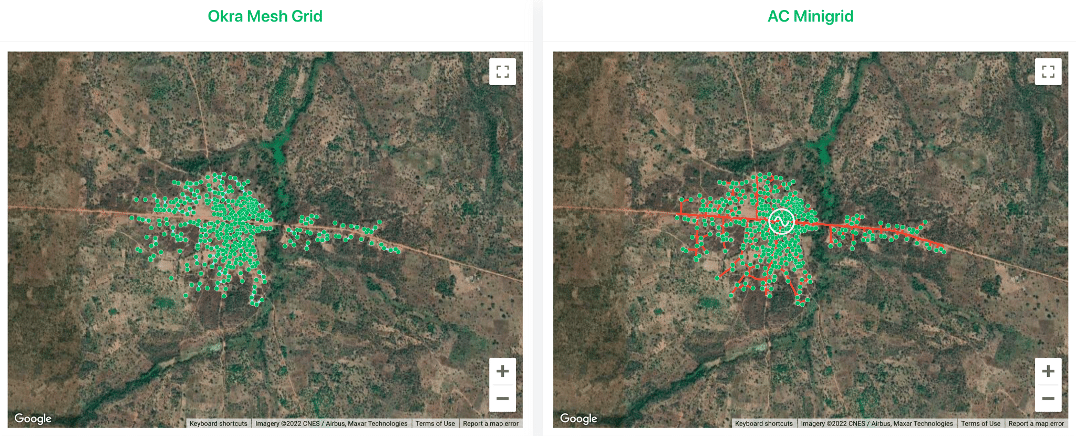
Fig. 3c — Automatically generated distribution mappings of a mesh-grid and mini-grid. The increased size of this village means that the mini-grid would likely require three-phase distribution rather than single-phase as in the previous example.
- # households: 393
- Area: 0.78 km2 (503 households / km2)
- Avg. daily load: 250Wh
- Simultaneous avg. peak load: 150Wp
- Cost comparison: ~$591/cxn for mesh-grid vs ~$670/cxn for mini-grid
As we move to a village with several hundred homes, the mini-grid remains competitive in price at this energy and power rating (see details above). Consequently, this could be seen as more of a grey area in the choice between a mesh-grid and mini-grid because the larger scale of this community makes a mini-grid a more compelling infrastructure solution than in the previous, smaller site.
But what happens if the community needs more energy in the future? Running the simulation again but with increased energy/load capacity, we get the following:
- # households: 393
- Area: 0.78 km2 (503 households / km2)
- Avg. daily load: 250Wh
- Simultaneous avg. peak load: 600Wp
- Cost comparison: ~$784/cxn for mesh-grid vs ~$1130/cxn for mini-grid
The increase in power rating for the mini-grid requires MV distribution, which causes the overall mini-grid cost to increase far beyond just the additional generation and storage capacity. In contrast, the mesh-grid distribution costs remain exactly the same, and so the only additional cost is the result of adding more panels and batteries. This means that the energy capacity of a mesh-grid can be incrementally scaled up over time without requiring any invasive changes to the infrastructure, allowing for both a low initial cost and a smooth path to scaling energy consumption.
On the other hand, mini-grids hold value as a lasting infrastructure investment, opening up the future possibility of bidirectional power sharing with the main grid, should it be extended to reach the mini-grid. While this possibility is appealing, in the majority of cases, the main grid will remain financially unviable to extend. In those cases where the main grid does reach these communities, it can also connect to a mesh-grid using our solution called the Grid Gateway. Effectively, it is an AC to DC converter that diverts excess power from an AC source into a mesh-grid, leveraging the low-cost distribution of the mesh-grid and future-proofing the mesh-grid assets.
Another possible solution would be a hybrid of the two technologies, where a mini-grid is used for the most dense portion of the community as well as any commercial loads and a mesh-grid is deployed for the remaining, using the Grid Gateway to send excess power from the mini-grid into the mesh-grid.
Large community, medium voltage mini-grid – Bassa
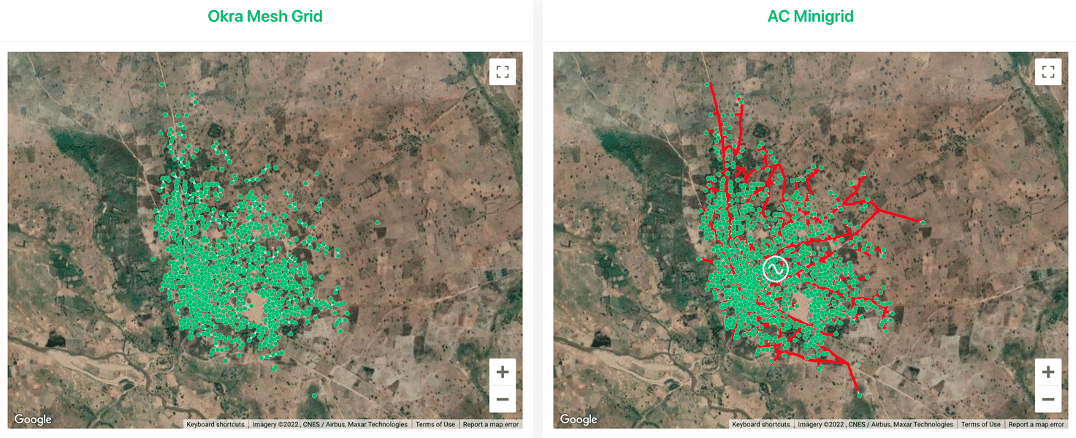
Fig 3d. — Automatically generated distribution mappings of a mesh-grid and mini-grid. The increased size of this village means that the mini-grid would likely require three-phase distribution rather than single-phase as in the previous example.
- # households: 987
- Area: 1.82 km2 (542 households / km2)
- Avg. daily load: 250Wh
- Simultaneous avg. peak load: 150Wp
- Cost comparison: ~$594/cxn for mesh-grid vs ~$780/cxn for mini-grid
In this village, the mini-grid would require MV distribution even at 150Wp because of the number of households and spread of the village. This causes the cost of distribution to shoot up, making a mini-grid significantly more costly than the mesh-grid, even though the cost difference is significantly reduced (compared with the previous example) due to the larger scale of this village.
The cost of MV AC distribution means that mesh-grids may actually be an effective solution for these larger sites – villages with more than a few hundred households, but without the sheer size or energy demand to make the investment in MV distribution pay off. This contradicts the common notion that mesh-grids are only suitable for exceptionally sparse and/or isolated sites. The counter also holds true – if this mini-grid could be built around a commercial load such as a telecom tower or industrial plant, or if the site was already connected to existing distribution, then the additional cost of AC distribution may be justified.
As in the previous example, a hybrid option could be used to leverage the strengths of both solutions. For instance, a mini-grid could be used to power the most dense part of the community as well as high power commercial loads with excess power being shared into a lower cost and more nimble mesh-grid in order to reach every home in the village.
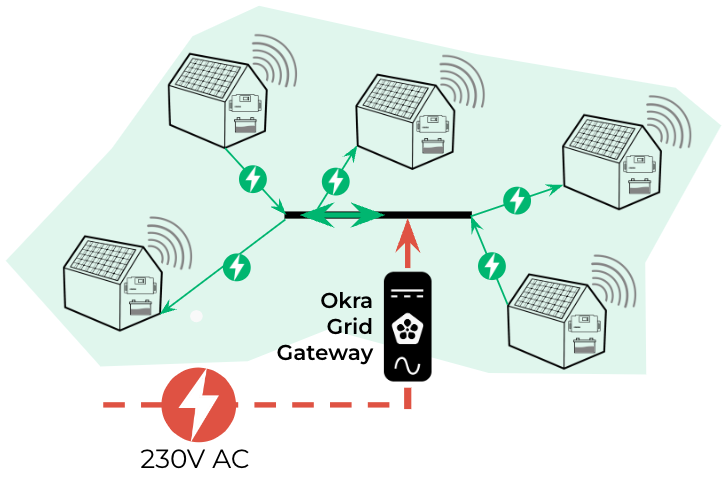
Fig 4. — An example of a hybrid mini-grid ←→ mesh-grid interface via Okra’s Grid Gateway solution
Mesh-grids prove to be a versatile energy solution
By mapping distribution lines and physically modelling the AC mini-grid distribution constraints, Okra’s Network Planner has allowed us to push the boundaries of our knowledge regarding the application of mesh-grids and mini-grids.
We find that the largest differences in cost come from the differences in distribution infrastructure. Mesh-grid distribution is a relatively small and nearly constant cost while the cost of AC distribution increases dramatically with the scale of energy capacity required. In practice, as in our analysis, this means that mini-grid viability can be realised in a few distinct scenarios:
- Extremely low power: ~100W per household, single phase distribution in a densely populated community – in this case the cost of the mini-grid is only slightly higher than the mesh-grid as we saw in the second example site; but mesh-grids would still be a cheaper, faster and lighter solution in this scenario.
- Extremely high power: centred around commercial anchor loads and/or exceptionally dense and large community – in this case the cost of higher power AC distribution could be justified by the energy demand of the community, but these communities make up only a small proportion of the off-grid space. A hybrid mini-grid and mesh-grid solution may be optimal to electrify the entire community including clusters on the edge of the densely populated centre.
- Main grid connection: if the main grid arrives, a mini-grid can be smoothly integrated to use two-way power sharing – in this case the existing mini-grid infrastructure can provide further value to the community through this interconnection. This possibility would typically be limited to communities within reach of the main grid.
Thus, mini-grids maximise their value for dense communities with exceptionally high energy demand, or that may be connected with the main grid in the near future. However, main grid connection is a rare occurrence in practice, and the vast majority of off-grid communities lack the energy demand to make a higher power mini-grid worth its high cost.
On the other hand, mesh-grids represent a more scalable solution for residential off-grid electrification. As the scale of energy demand and community size increases, the cost per connection of the mesh-grid remains roughly the same, while the cost/cxn of the mini-grid grows rapidly until we reach MV distribution – at which point it takes a huge amount of energy demand to justify the investment.
Therefore, we believe that the mesh-grid presents a more cost-effective solution for the majority of off-grid sites – all the way from the smallest communities up until the most energy dense, which may be able to offset the cost of an MV mini-grid.
As a final note, we know there are people out there who make a living designing mini-grids.
We encourage them to comment on this, and share their thoughts and lessons. We’re always improving our algorithms, and we’re keen to learn how we can make this network planning tool more valuable for the industry going forwards.
At the end of the day, we’re all striving to increase energy access across the globe – and data-driven insights are key to achieving this. The challenge lies in ensuring that our simulation and modelling methods are truly indicative of what happens in reality.
Please use this form to leave feedback on this blog. You can let us know if we’ve missed anything, or if you would like to try out the Network Planner yourself!
Appendix
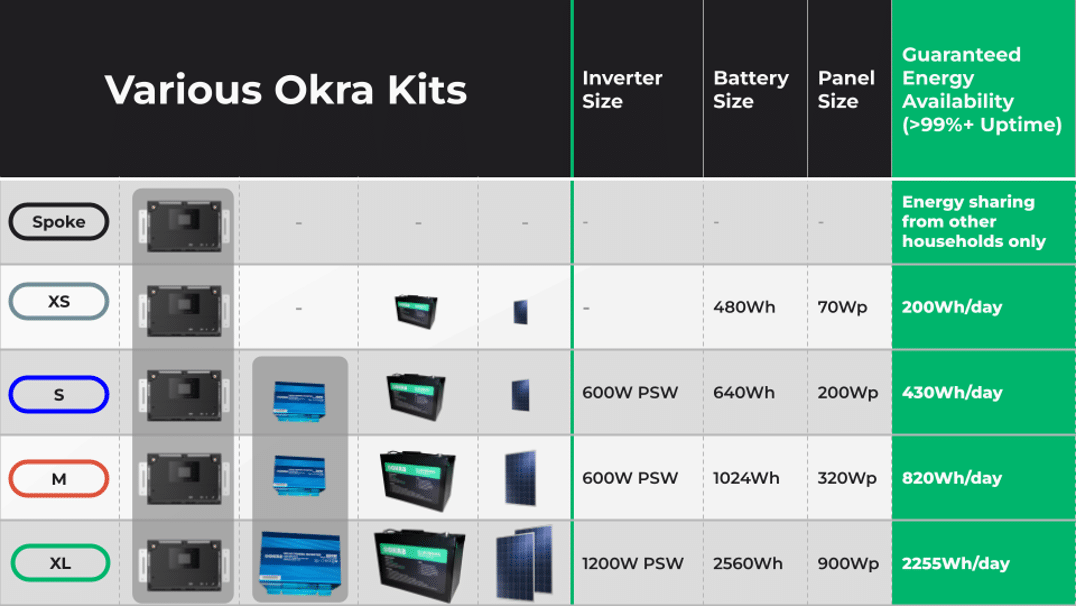
Figure 1b – Detailed breakdown of each mesh-grid kit.
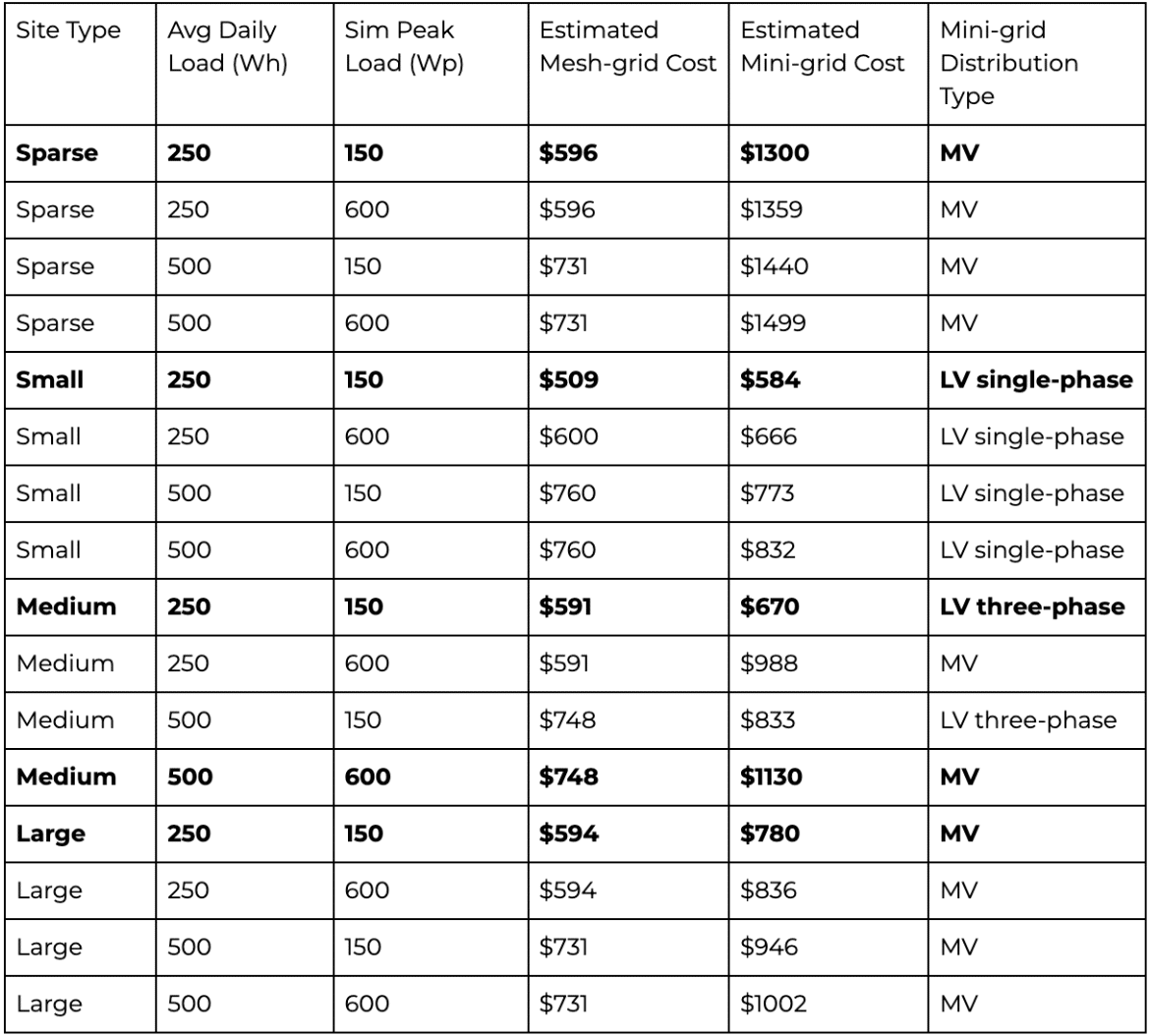
Breakdown of estimated costs for mesh-grid and mini-grid over the sites pictured in this article at different power and energy ratings. Bold entries are discussed in the article.